Insight: Barraud’s Weight and the Marine Chronometer
Solving the middle temperature error.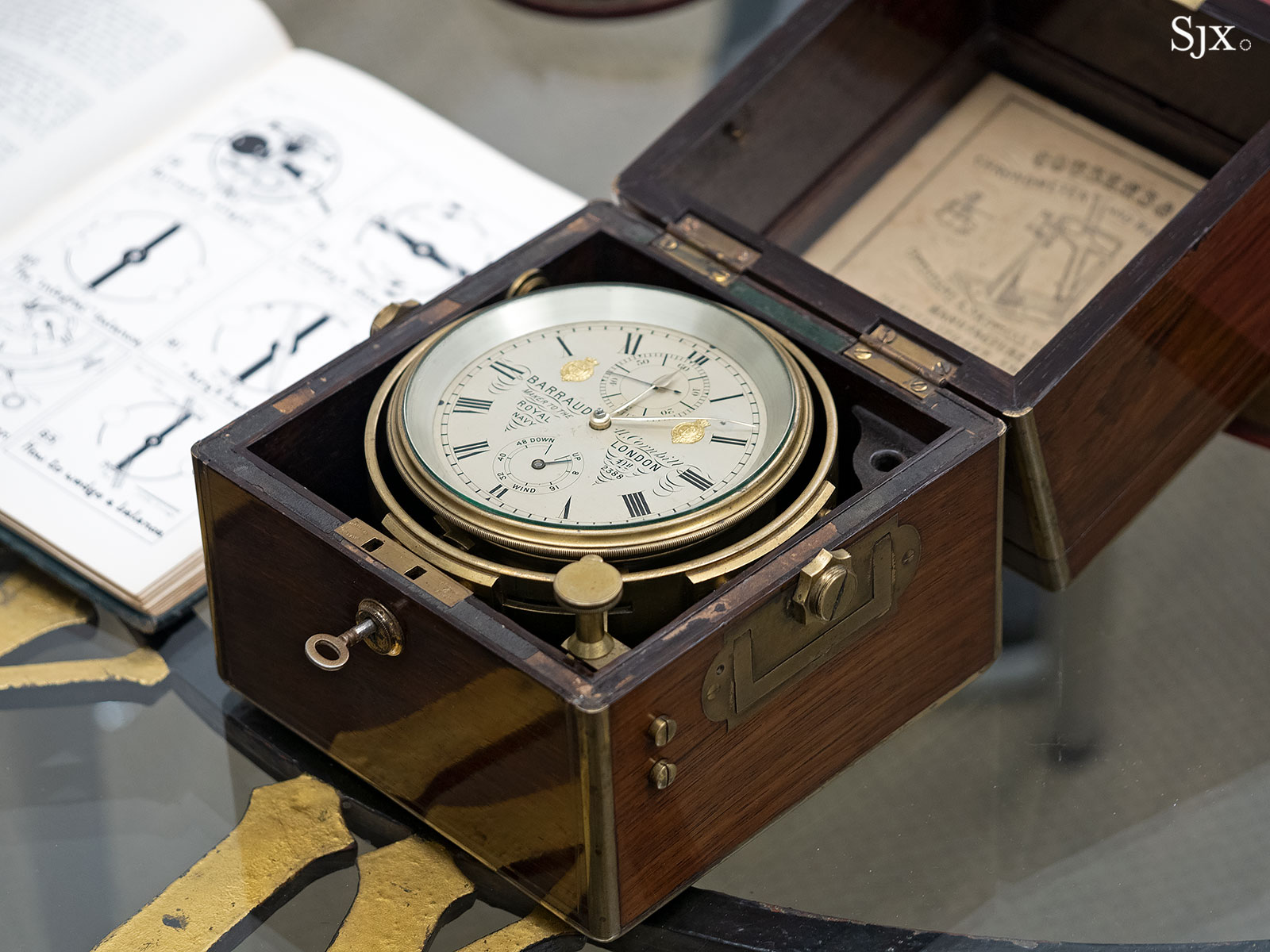
Although precision watchmaking is today mostly synonymous with Switzerland, the oft forgotten truth is that much of the technological progress and development in horology during the 18th and early 19th centuries originated in England. The preeminence of English watchmaking during that period is perhaps personified by John Harrison, the carpenter-turned-clockmaker best known for his invention of the marine chronometer.
Harrison and his contemporaries advanced horology in an era where the pursuit of accurate timekeeping was of national importance for the United Kingdom: the marine chronometer enabled ships to traverse the highs seas while maintaining positional accuracy over extremely long distances, an achievement that no doubt helped the rise of the globe-spanning British Empire.
The curious chronometer
On a recent visit to Charles Frodsham & Co Ltd. – the English chronometer manufacturer now run by Philip Whyte and Richard Stenning that’s making wristwatches equipped with a natural escapement – a rather curious timekeeper from England’s heyday as a watch and clockmaking nation emerged.
Dating to 1845, it was marine chronometer no. 2388 manufactured by Barraud, later Barraud & Lund, a now defunct English watchmaker (though a descendant of the company, Lund & Blockley, remains in operation to this day as a retailer in Mumbai). While the well-preserved state of the clock was impressive in itself, closer inspection of the movement revealed a peculiar feature.
Sitting on the balance wheel rim is a largish, round weight that doesn’t look like it should be there as it is mounted on only side of the balance. A conventional balance wheel is typically symmetrical, and, well, balanced. More intriguingly, the circular weight is actually a container for an intricate mechanism within that corrects for temperature variations that affect timekeeping – specifically, the phenomenon known as the “middle temperature error”.
But to further understand the significance of the unusual device on the balance, we have to explore why the marine chronometer came to be, starting when civilisation developed the means to travel long distances across the oceans.
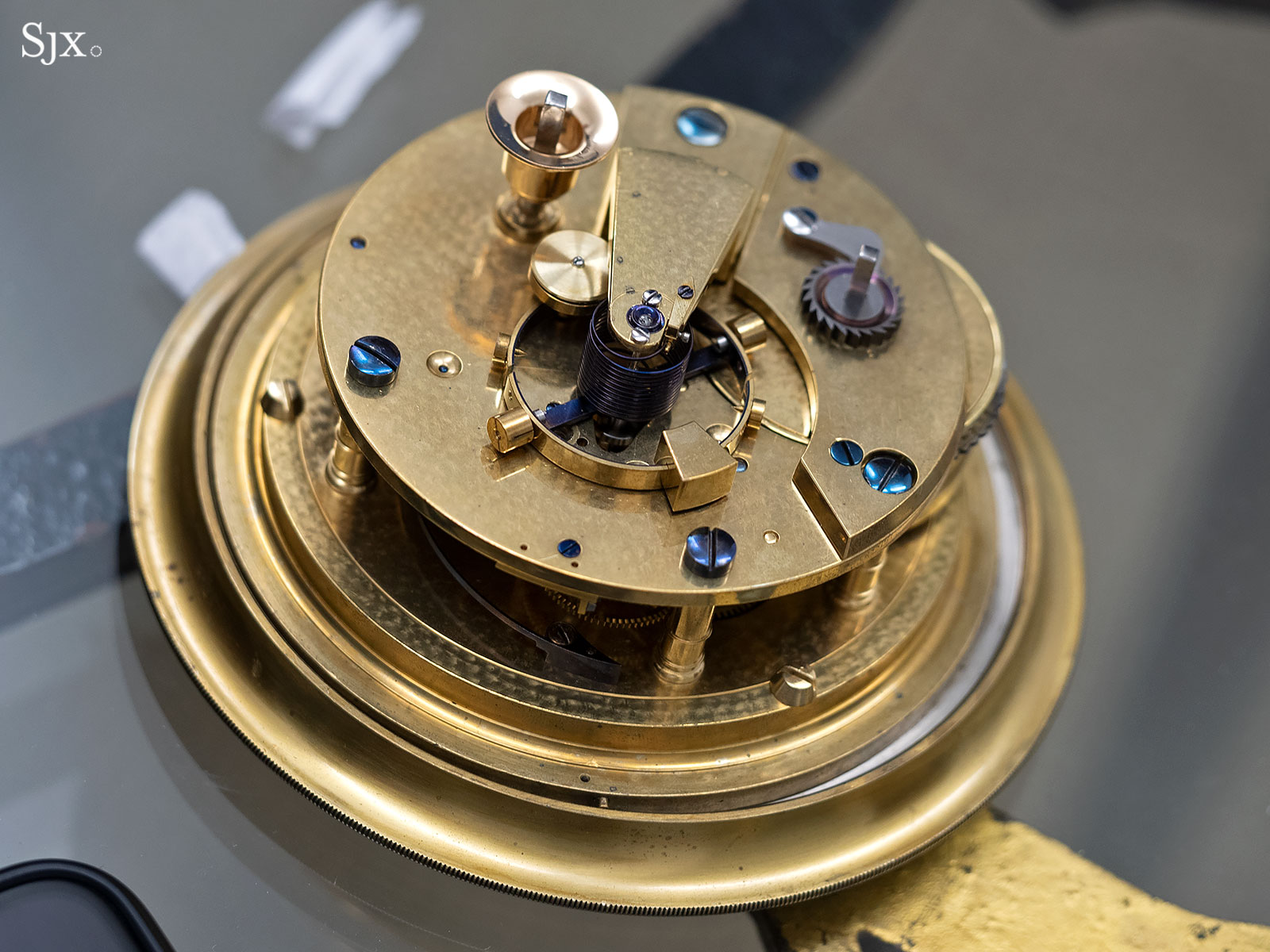
The movement of Barraud marine chronometer no. 2388
Lost at sea
Prior to the invention of modern navigation technology such as GPS, an accurate timekeeper was paramount to navigation at sea. To illustrate its relative importance, one has only to look at the cost of a marine chronometer. While marine chronometers initially cost several hundred pounds, by the mid 1800s a good example cost £20, but a ship usually carried three as standard.
The HMS Beagle, famed for carrying Charles Darwin around the world, was finished in 1820 for a little under £8,000, and then rebuilt in 1830 for almost the same amount. That meant the Beagle’s timekeepers cost a significant amount relative to the total cost, about 1% of the whole ship. The comparison is inexact, but in modern terms that would be equivalent to a $1 million timekeeper on a $1 billion guided-missile frigate.
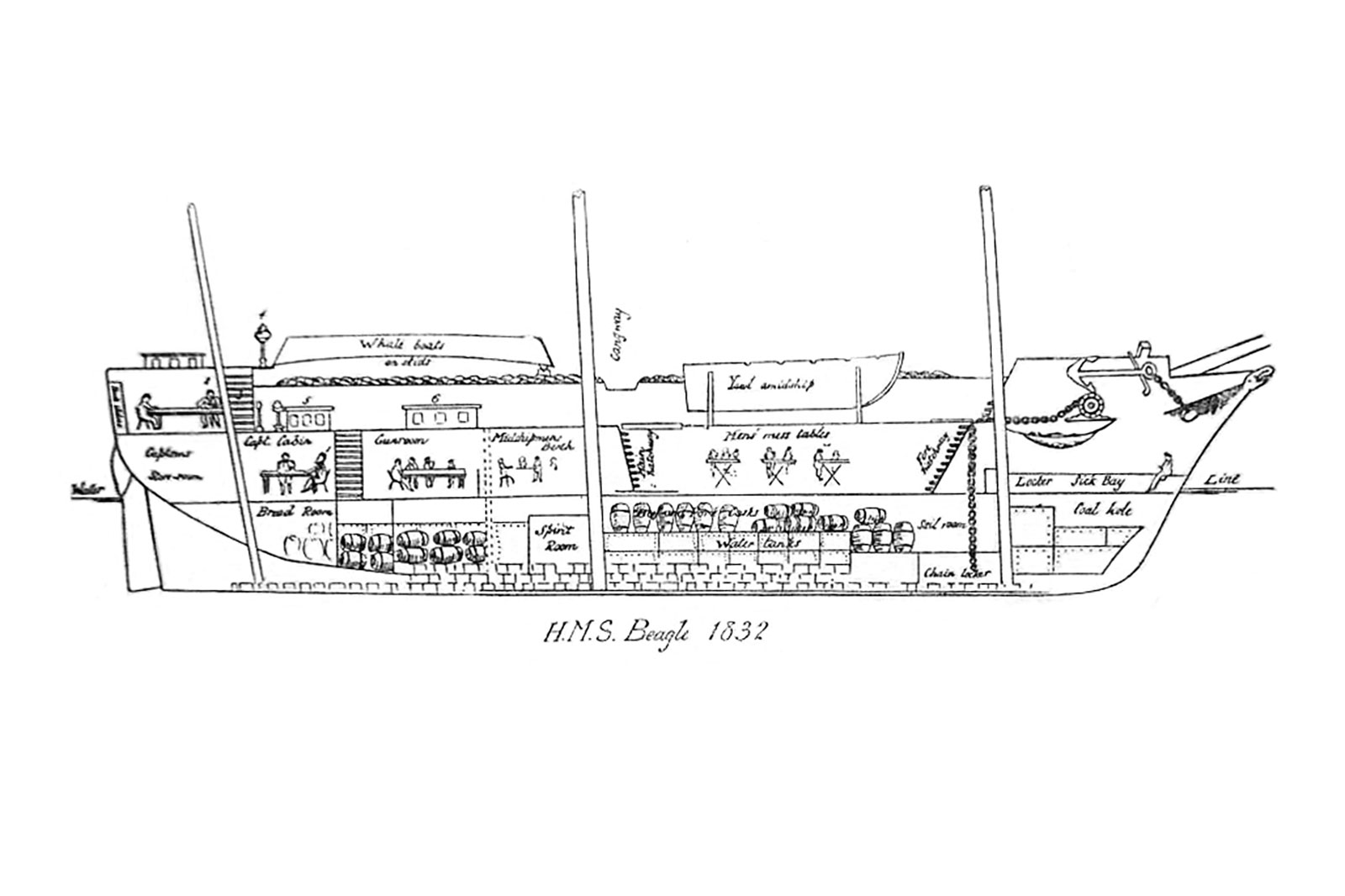
An 1832 drawing showing the longitudinal section of the HMS Beagle. Image – Wikipedia
What made such a small piece of equipment so important? Put simply, the marine chronometer was the practical and reliable method of determining longitude during long sea voyages. Being able to correctly ascertain a ship’s current position was especially important, as any miscalculation would result in the risk of missing the target destination, by up to hundreds or thousands of miles, and being lost at sea with dwindling supplies.
So determining a ship’s position was a grave matter for the country during an age of colonial expansion. Widely known as the Longitude problem, maritime navigation was so crucial that in 1714, the British government offered a reward of £20,000 for anyone that could solve it, or more specifically, build it. The solution to the problem was already known – an extremely accurate clock – but none of the portable clocks available at the time were up to the challenge.
Celestial navigation
A clock’s relation to navigation is simple. For millennia, sailors had to look to the skies to determine their position, being surrounded by endless water with no landmarks. Known as celestial navigation, this technique involved using the sun, moon and stars as signposts in the sky. When executed precisely, celestial navigation can be used to pinpoint the vessel’s latitude and longitude with a good degree of accuracy.
Latitude determines the north-south position on Earth – the higher the latitude, the closer the geographic North Pole, and vice versa. A magnetic compass indicates the general direction of the poles, but it doesn’t indicate latitude, which is the distance from the poles.
Thankfully, a solution exists in the clear night’s sky in the Northern Hemisphere. A prominent star known as Polaris, or the North Star, sits stationary, regardless of the observer’s position on Earth. All the other stars appear to slowly travel as time passes due to the Earth’s rotation, except Polaris, making it something of a celestial beacon.
Measuring the angle between Polaris and the horizon indicates the observer’s latitude, a simple and powerful tool for navigation. The Southern Hemisphere, however, doesn’t have an equivalent of Polaris, so constellations such as the Southern Cross are used as the fixed reference point for latitude measurement.
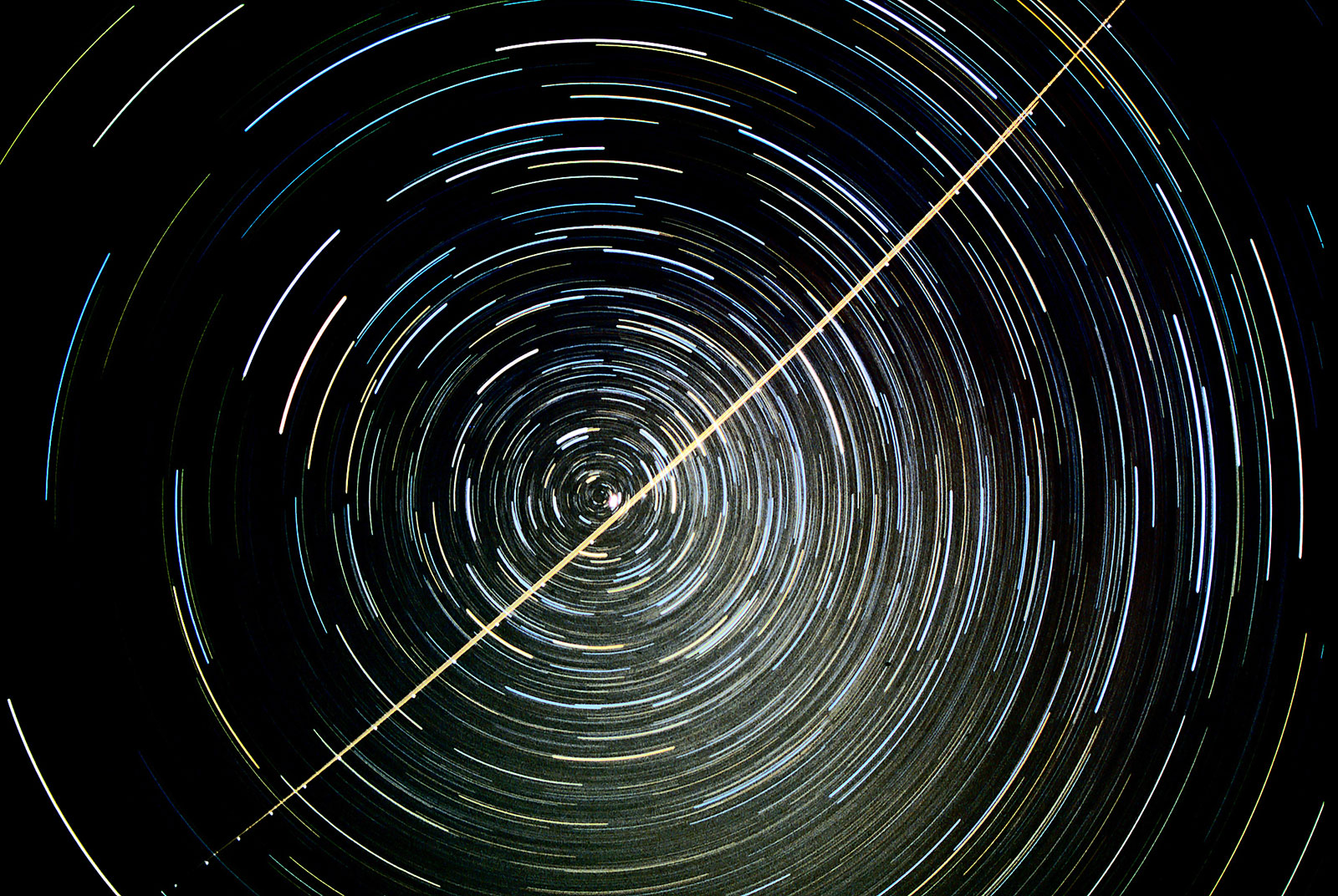
A star trail photo from the northern hemisphere, with Polaris visible as the centre bright dot. Image – Wikipedia
But determine the exact position of a ship, longitude – or east-west position on Earth – is also necessary (and longitude also dictates the time zone). Unlike latitude, however, determining longitude solely via celestial observation is a complex undertaking. Astronomers long possessed the solution, but the solution required an instrument that had yet to be invented.
That instrument was the marine chronometer. Built to keep perfect time despite the elements, the marine chronometer was impervious to the waves rocking the ship, or the drastic temperature fluctuations from day to night on the high seas.
Despite being a portable clock in a box, a marine chronometer required a surprisingly elaborate process to set up. Before a ship set sail, the chronometer was set to home time. Correct setting was ensured by using the sun as a reference: observing the sun at its highest point in the sky, the definition of solar noon, or 12:00 pm. (Solar noon doesn’t precisely coincide with the civil time of clocks used in daily life, so a correction factor, known as equation of time, was required.)
Once set, the chronometer was wound regularly, but its time setting left untouched. As the ship travelled to a different longitude, its time zone effectively changed, but the chronometer remained on home time. Thus, by observing local solar noon at the ship’s current longitude, a sailor could determine how far east or west was located in reference to the time displayed on the chronometer.
For instance, if the sun was observed at the highest point in the sky while the clock read 9:00 am, it could be deduced that the ship was three hours ahead of the departure port, which meant longitude of 45° eastward.
Ultimately, by combining the two techniques to determine latitude and longitude, a ship’s position could be determined with a good degree of accuracy. Of course, all of that hinged on the marine chronometer being spot on throughout the journey.
The function of a marine chronometer was so vital that nothing could be left to chance – so a ship carried three of them just to be sure. A single marine chronometer could be wrong, while a pair would render it impossible to determine which one was correct. With three the task was easy: if one happened to gain or lose time, the other two clocks could be used to determine the correct time and adjust the offending clock.
Of gravity and temperature
Unusual for its time, the construction of the marine chronometer arose from the need it was designed to fulfil. Conventional clocks of the era were designed to keep time with a pendulum that swung back and forth at a precise rate of one second per cycle, resulting in the characteristic “tick” and “tock”. The use of a pendulum meant that the clock’s operation was dependent on gravity.
As a result, the orientation of a clock was important. That was a moot point in a home or office, where a clock would sit stationary on a mantelpiece or desk. In contrast, a clock on a ship was tossed around by the ocean, causing the clock to tilt off the vertical, resulting in gravity pulling the pendulum sideways, and the possibility of the clock stopping altogether. An alternative oscillator was necessary to make a ship-borne chronometer viable.
This led to the development of the hairspring and balance wheel – invented by Robert Hooke and Christiaan Huygens separately – now the predominant regulator in mechanical wristwatches. A coiled spring attached to a weighted rim that swung back and forth, the invention allowed for in a compact timekeeper that was less sensitive to gravity effects, allowing it to supersede the pendulum.
The balance wheel, however, possessed intrinsic flaws as a result of the materials of the era. At the time, balance springs were made of carbon steel, which is temperature sensitive. At higher temperatures, the alloy softens, resulting in the hairspring losing stiffness and the clock running at a slower rate. The magnitude of rate error was up to ten seconds per degree – a potentially massive variation considering the change in temperatures at sea between day and night, or in inclement weather.
The problem was first solved in 1753 when John Harrison invented the bimetallic balance curb, which compensated for temperature-induced changes in the hairspring. Around a decade later, a simpler solution was invented by Pierre Le Roy, who applied the same concept to the balance wheel itself. Known as the bimetallic balance wheel, this was constructed of two alloys: a strip of brass on the outer rim and steel on the inner side, with breaks at two points on the rim. And weights were affixed onto the arms of these curved strips for regulation.
As brass expands more than steel with an increase in temperature, the outer layer expands more than the inner layer, resulting in a greater curvature of the balance arms, causing the arms to contract. That leads to a decrease in the moment of inertia, causing the balance wheel to oscillate faster. The same principle lies behind a figure skater stretching his arms out in order to lower his rate of spin, and drawing his arms inward to increase the speed. Ultimately, the decrease in the balance wheel’s moment of inertia compensates for the softness of the balance spring.
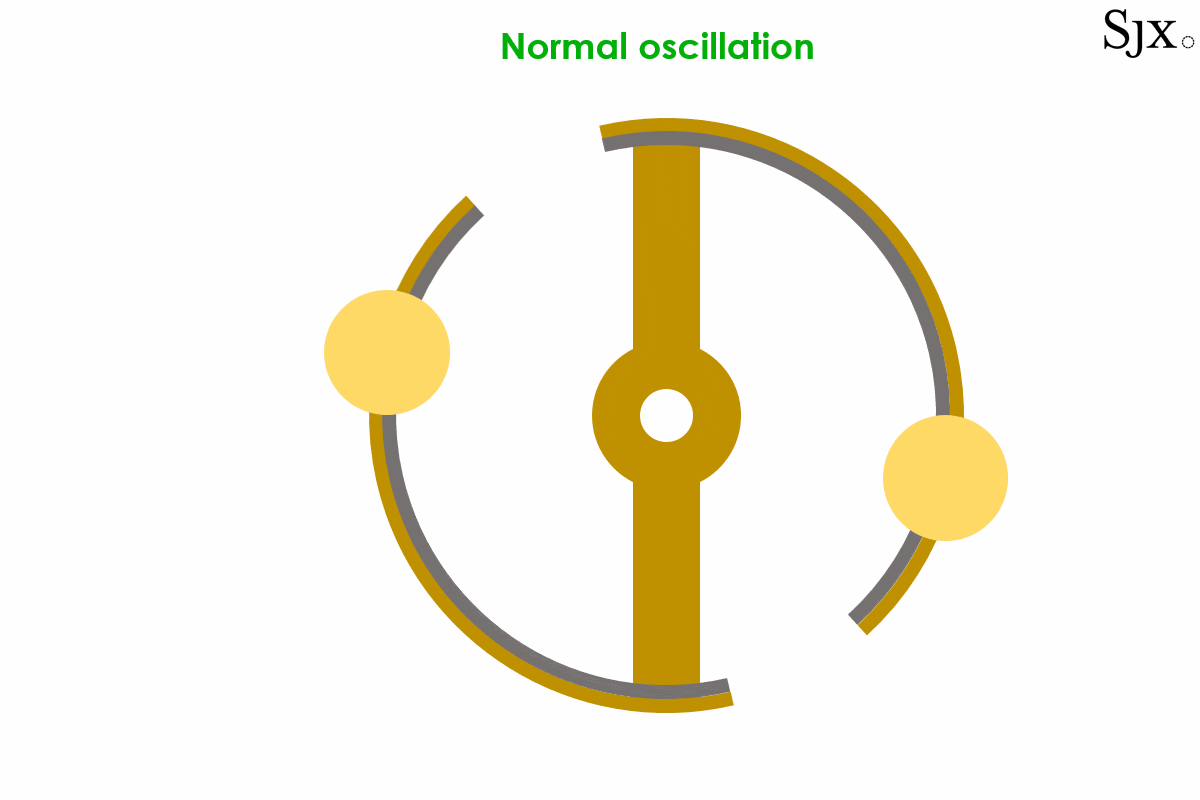
The function of a bimetallic balance wheel
The middle temperature error
The bimetallic balance wheel took care of most errors due to temperature changes. However, it was discovered in the late 18th century that a residual temperature error remained, a phenomenon known as the middle temperature error.
David Boettcher, a scholar and fellow of the British Horological Institute (BHI), had discussed this topic in comprehensive depth on his website. Put simply, it was observed that a properly-regulated marine chronometer with bimetallic balance ran run fastest at a peak temperature, while gradually losing time at a higher or lower temperature.
The cause of this error was widely debated, and generally attributed to the stiffness of a steel balance spring not varying linearly with temperature, but instead changing on a curve. As a result, the change in the stiffness of the balance spring is not perfectly compensated by the linear correction of the bimetallic balance, as exhibited in the animated graph below:
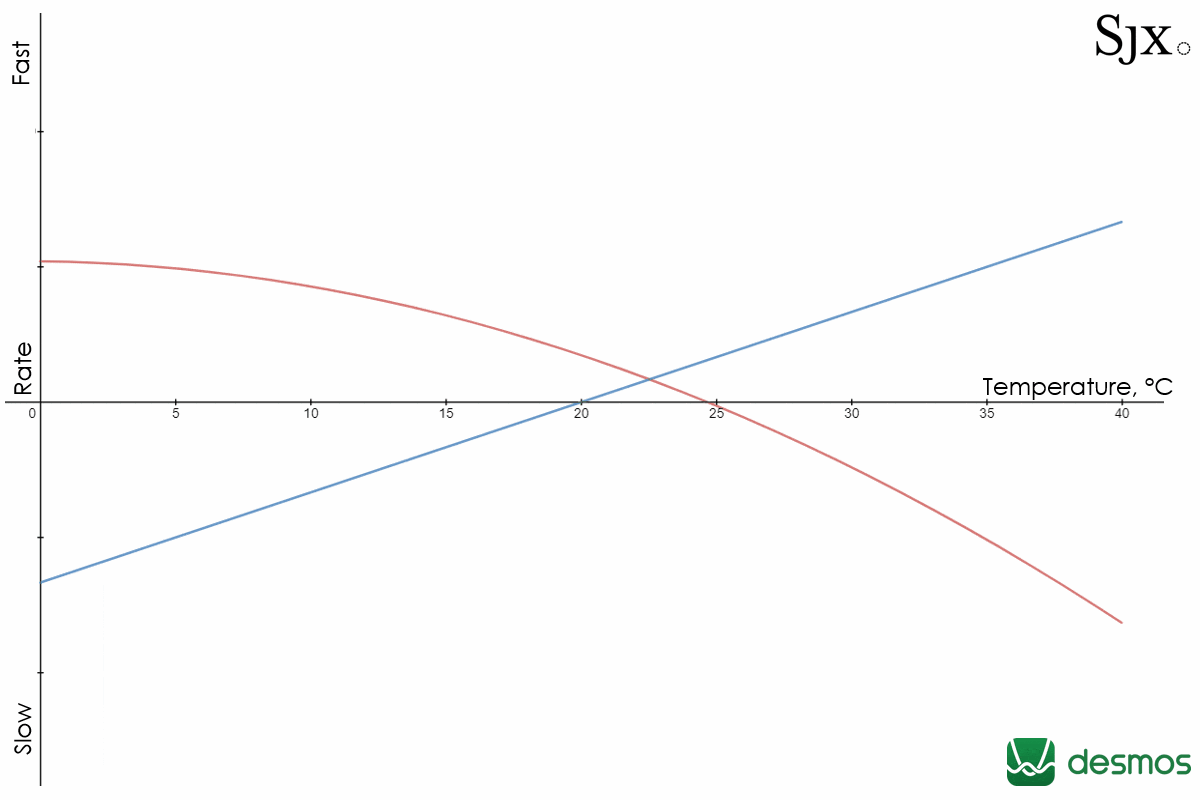
Bimetallic balance compensation of a steel balance spring, resulting in the middle temperature error
This leads to a deviation of a few seconds per day over the middle temperature range, which is much smaller than a non-temperature compensated balance wheel. But such a deviation, however small, would still accumulate over time. Preventing the deviation in the first place requires a compensation mechanism that mirrors the effect of rate loss from the balance spring, illustrated below:
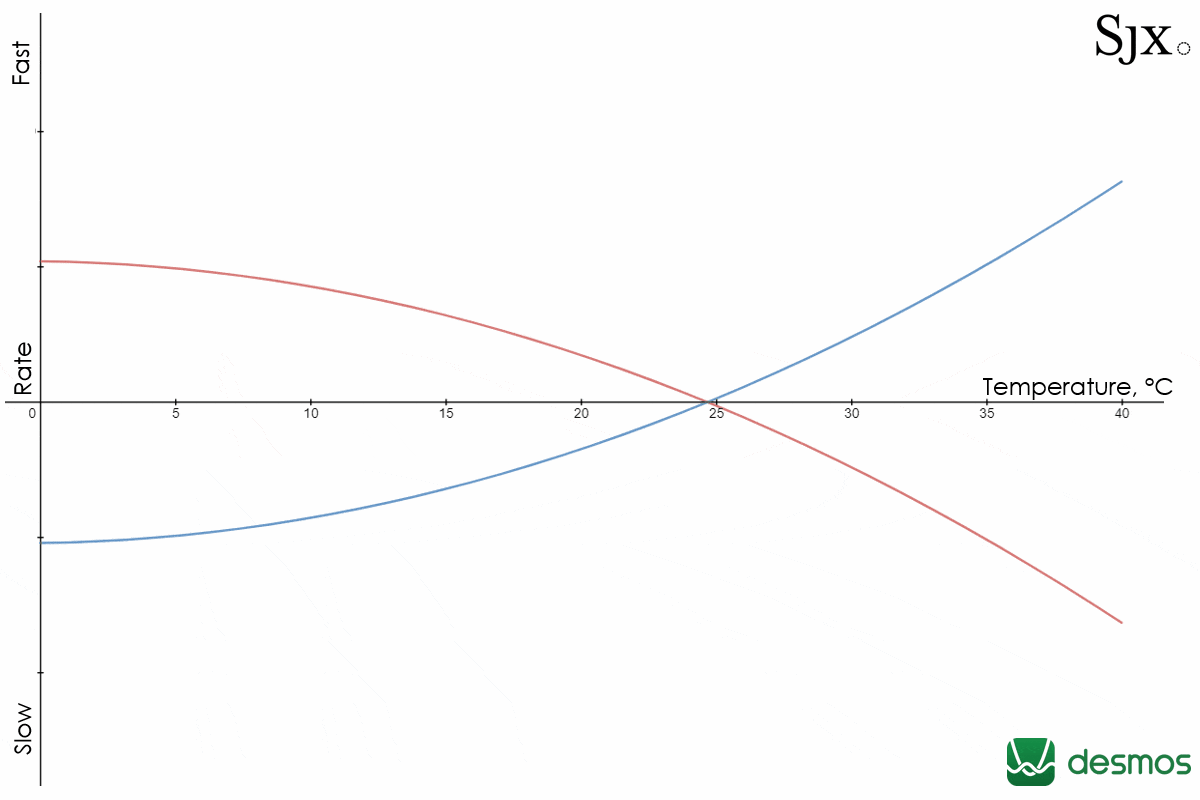
Ideal temperature compensation of a steel balance spring
Advancements in temperature-insensitive materials makes the topic a moot point in modern watchmaking. The Nivarox alloy that’s now standard for hairsprings has rendered the problem obsolete, even for the cheapest timepieces. But the middle temperature error was very real in the 19th century, leading to many attempted solutions, ranging from intricate mechanical solutions to alternative materials.
Barraud’s compensating weight
One solution, and definitely one of the most elaborate, was the compensating weight in the Barraud marine chronometer. Invented and patented in 1843 by John Richard Lund, who joined Barraud in 1838 and later became a partner in the firm, the weight sits on the rim of a bimetallic balance wheel, replacing conventional solid weights.
The marine chronometer owned Charles Frodsham is an excellent example of one equipped with Barraud’s weight. Curiously, on this particular marine chronometer, only one half of the balance wheel is equipped with a compensating weight, while the other has a standard wedge weight.
Like the bimetallic balance wheel it sits on, the weight itself is made of a temperature-sensitive alloy. Within the circular disc is an internal mass calibrated for the peak of the middle temperature error curve, which internally moves inward upon an increase or decrease in temperature. Drawing the mass inwards causes the balance wheel to speed up, which is the opposite effect to the middle temperature error and therefore compensates for it.
To explain the mechanism, Mr. Stenning unveiled a large-scale model of the weight, which details its internal mechanism:
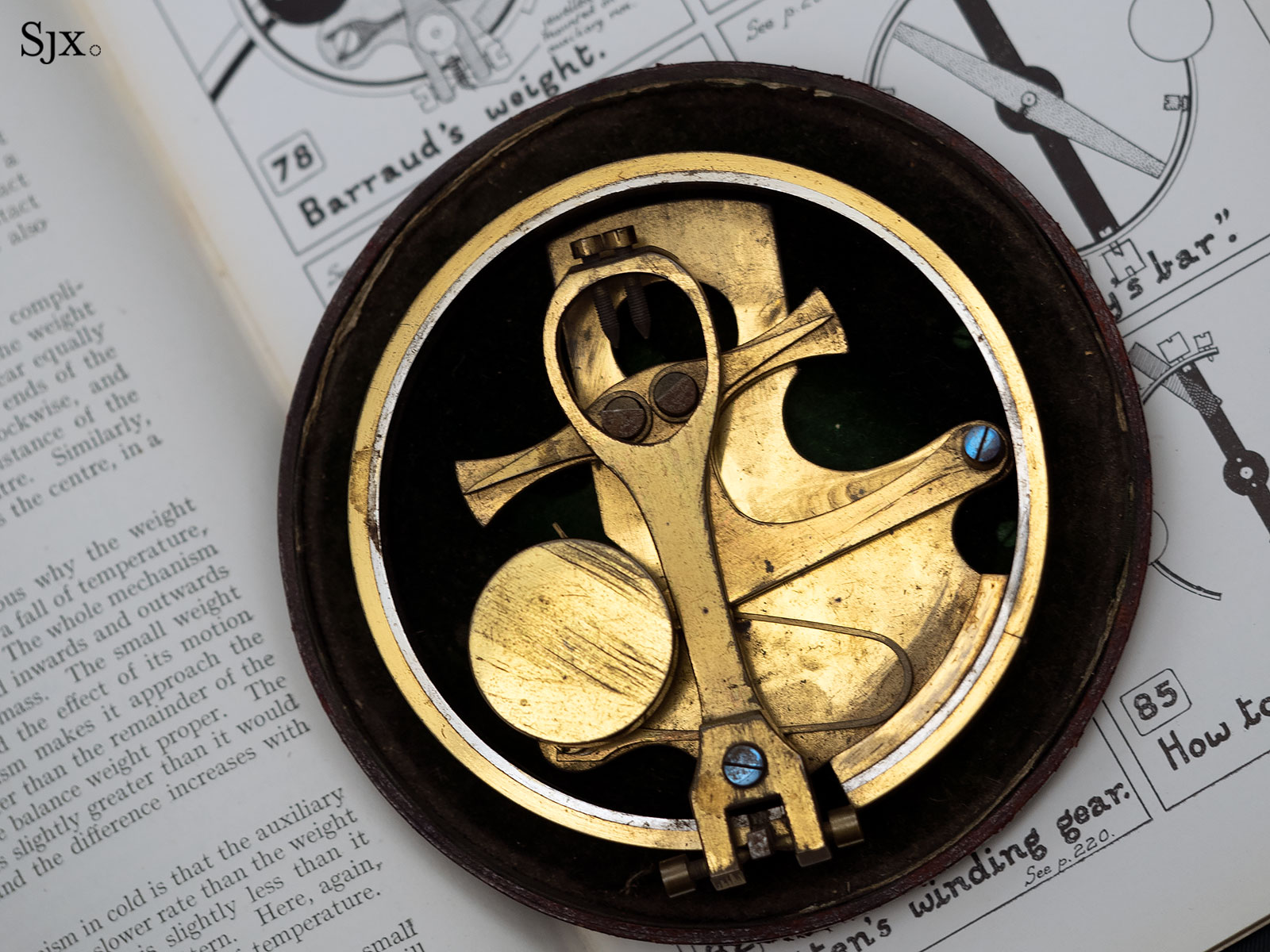
An enlarged scale model which demonstrates the inner workings of Barraud’s weight
The function of the compensating mechanism is illustrated below: the bimetallic strip (in green) expands or contracts upon a change in temperature, which drives an intermediate lever (in red) that pivots around point A.
On its opposite end, lies a pair of screws that rest against two inclined jewel planes. The planes are part of the lever (in blue) that holds the correcting weight of the device, and pivots at point B.
A restoring spring pushes upwards against the weight lever upwards, such that the inclined planes are always in contact with the screws.
When the intermediate lever pivots, the screws forcefully push against the inclined jewel planes. Due to the planes being in a wide “V” shape, the motion of the screws in both clockwise or counter-clockwise directions pushes against the planes, and therefore the weight lever. This redistributes the weight on the balance wheel rim, bringing it further inwards, thus reducing the overall moment of inertia and therefore causing a gain in time upon a change in temperature.
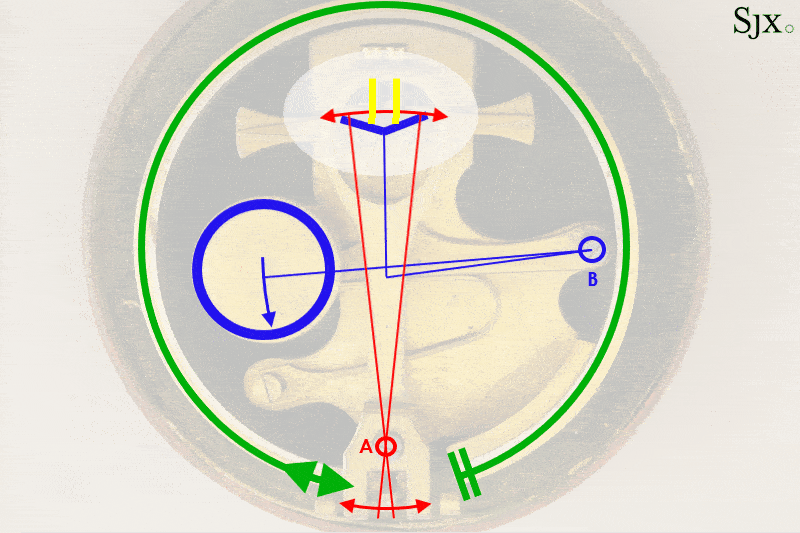
The animation illustrates how changes in the ambient temperature drive the mechanism
Barraud’s weight works in conjunction with the balance wheel itself; the bimetallic strips compensate for majority of the balance spring’s timing deviation, which was up to 10 seconds per day for every degree of temperature variation. Barraud’s weight, on the other hand, deals with the more subtle middle temperature effect, via an equally subtle shift of its own internal weight.
A finely-engineered timekeeper
Beyond the compensating weight, the Barraud marine chronometer is a sterling example of top-class English watchmaking in immaculate condition. These are some of its details clock, with brief annotations to explain the key elements.
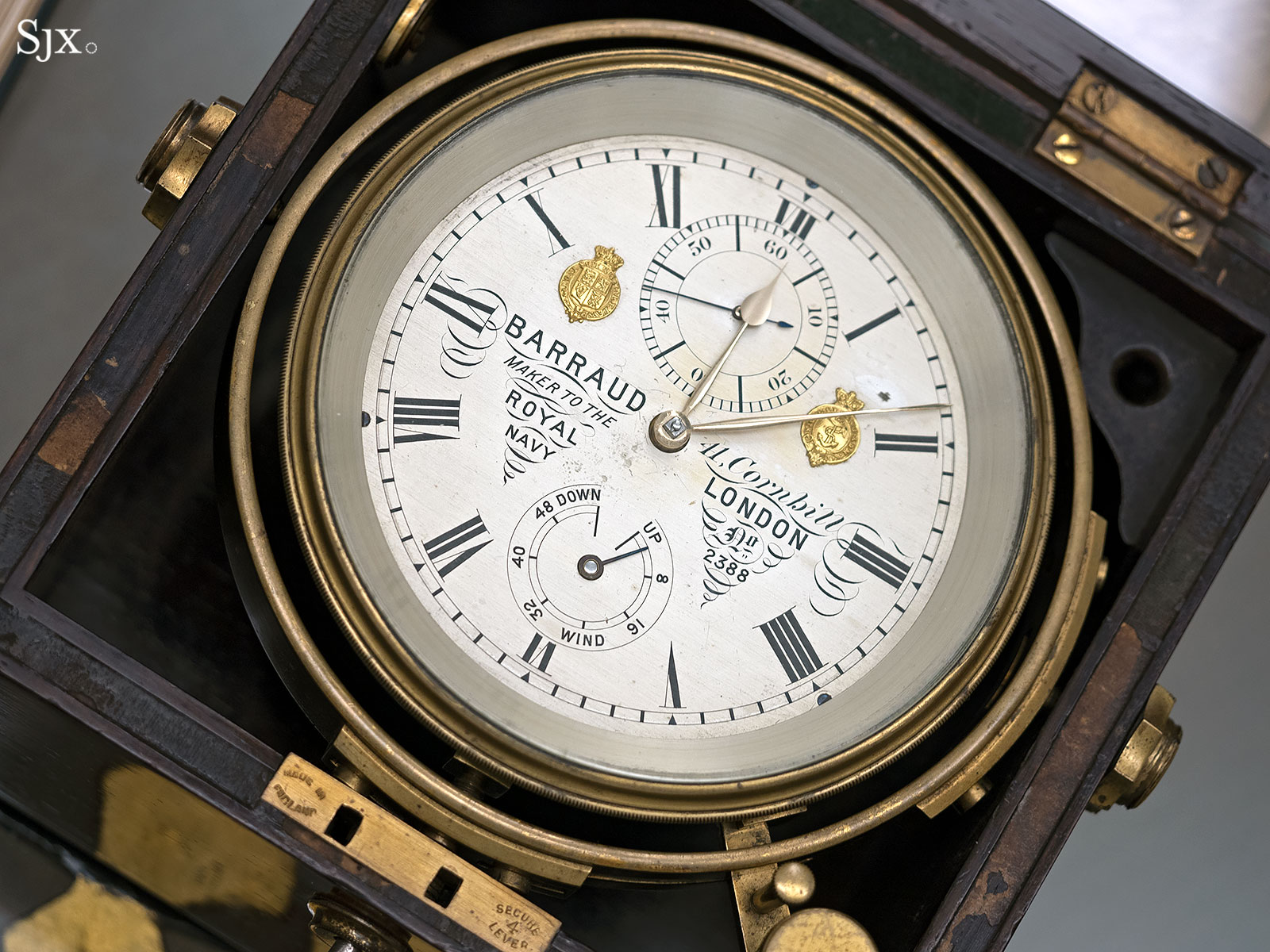
Barraud’s marine chronometer No. 2388, mounted on a gimbal within in a wood case. The gimbal ensures the clock remains horizontal relative to the surface of the earth, regardless of the motion of the ship, ensuring that the balance wheel is always oscillating in the ideal horizontal position and preventing any gravity-induced errors.
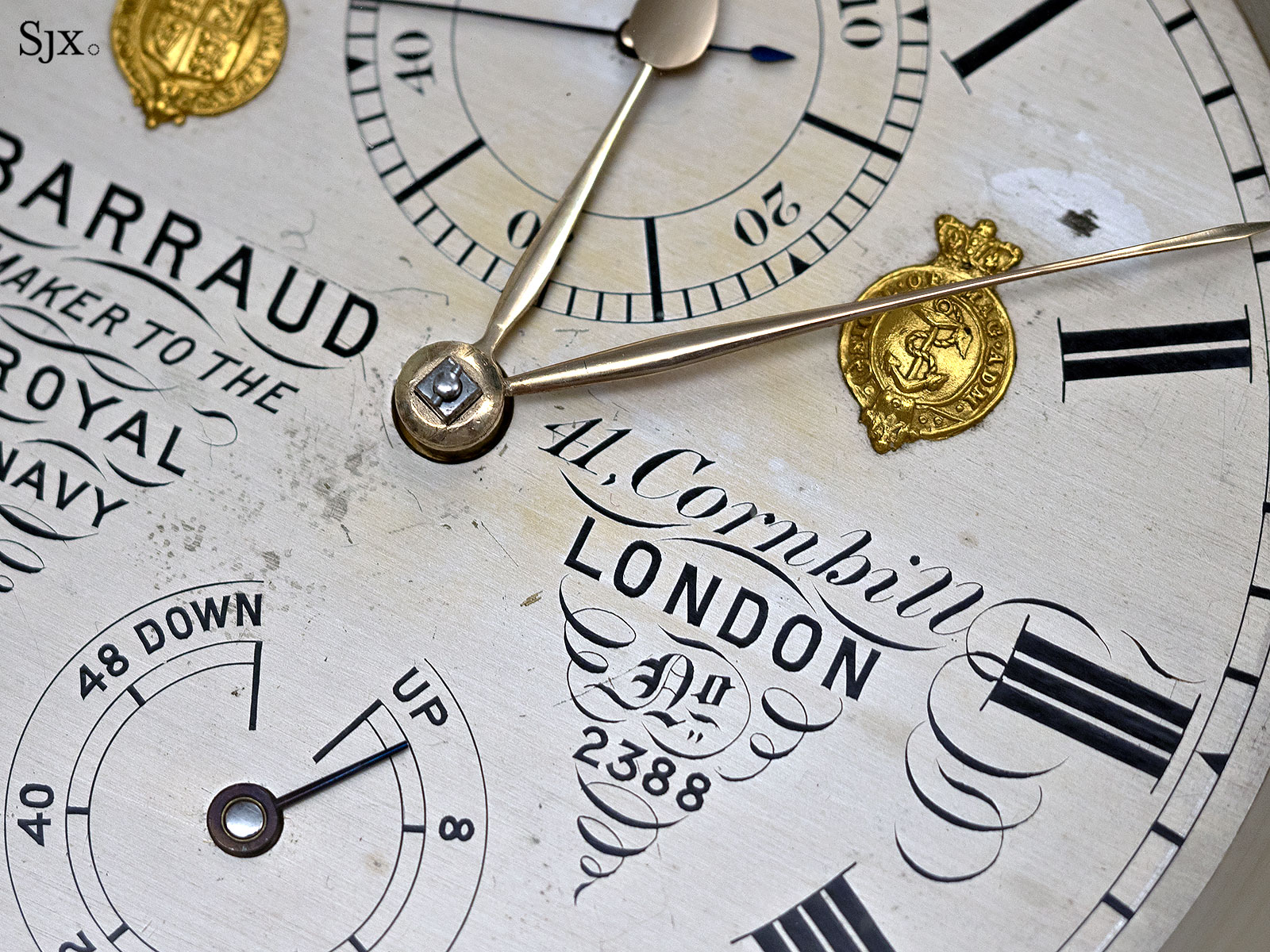
The dial is signed “Barraud, No. 2388, 41, Cornhill, London”. Above the signature is the applied gold coat of arms of The Admiralty, and at six o’clock is an especially important feature – the power reserve indicator. During a ship’s long voyage, it was paramount that the clock never ran out of power!
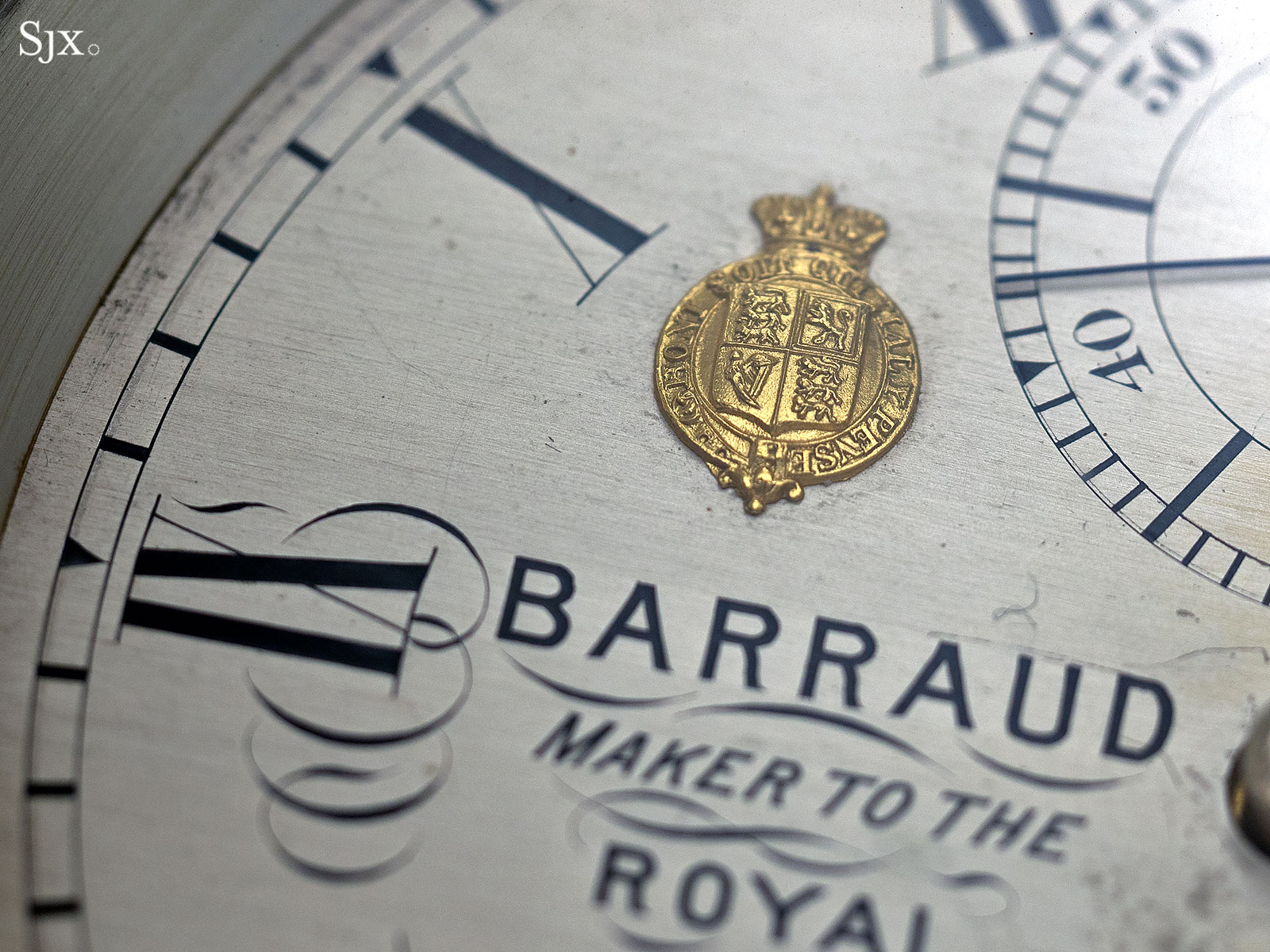
The applied Royal coat of arms of the United Kingdom sits at 10 o’clock. Also visible is the traditional silvered dial, with a linear-brushed finish and engraved, inked markings for legibility.
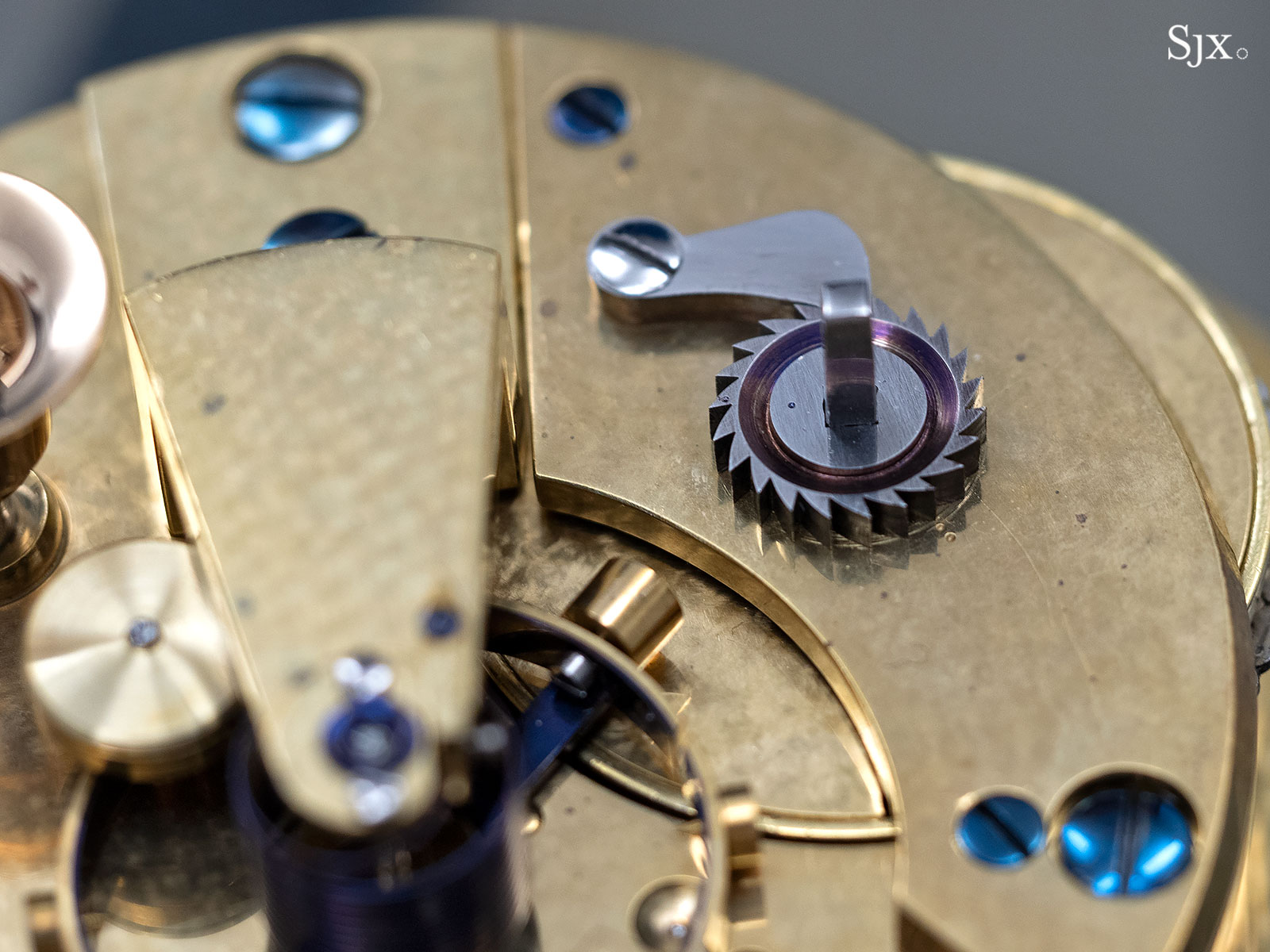
The winding click and barrel ratchet wheel fitted with a square arbor for winding the mainspring
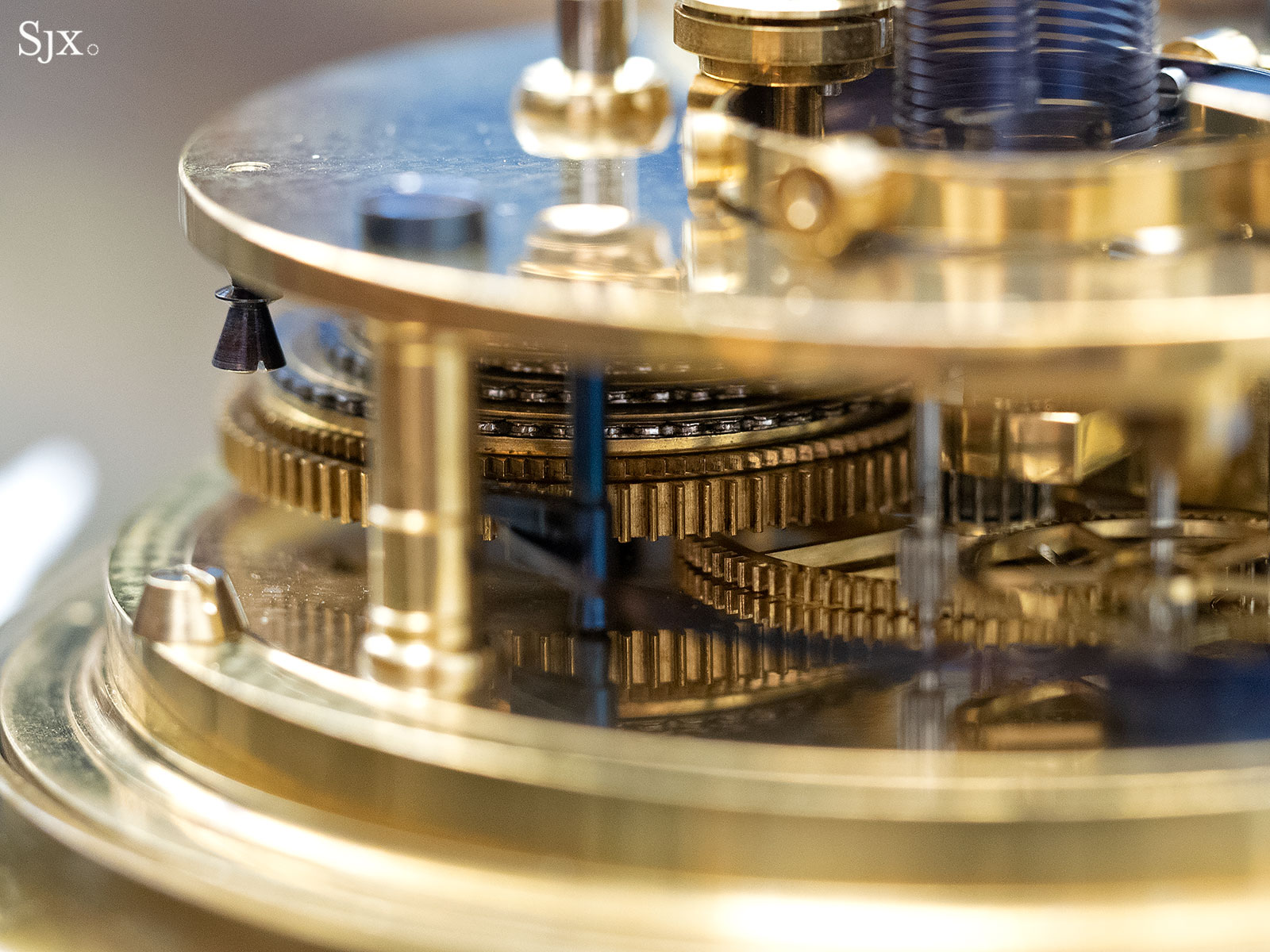
The two-day fusee and chain, sandwiched between the movement plates, which ensures a constant force is delivered to the escapement, which was especially important for a split bimetallic balance wheel that is susceptible to centrifugal forces as torque varies when the mainspring is fully wound or nearly empty.
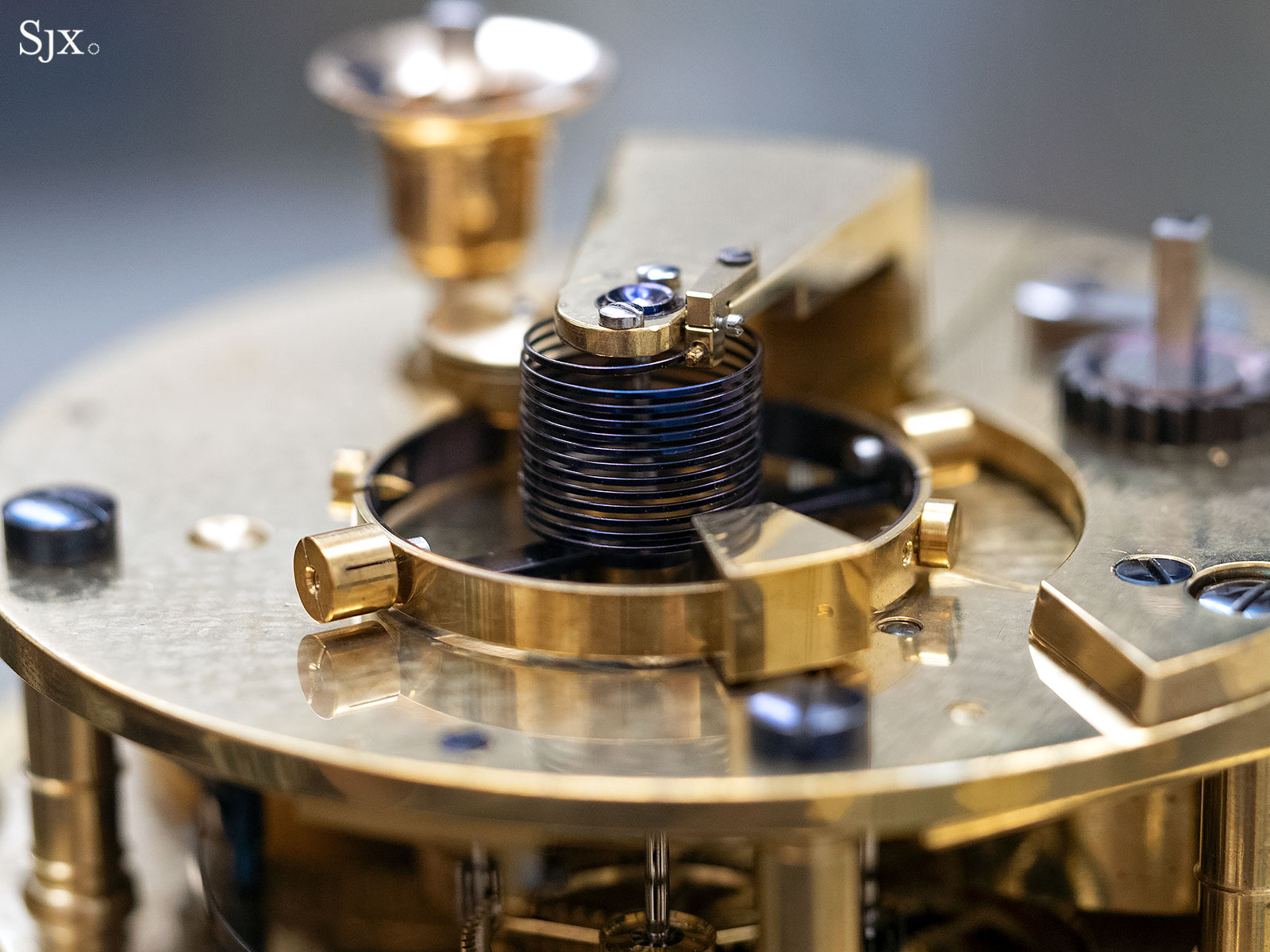
The tall, cylindrical balance spring for the balance wheel that was invented for ideal isochronism. The spring is made of carbon steel that is heat tempered, resulting in its blue colour.
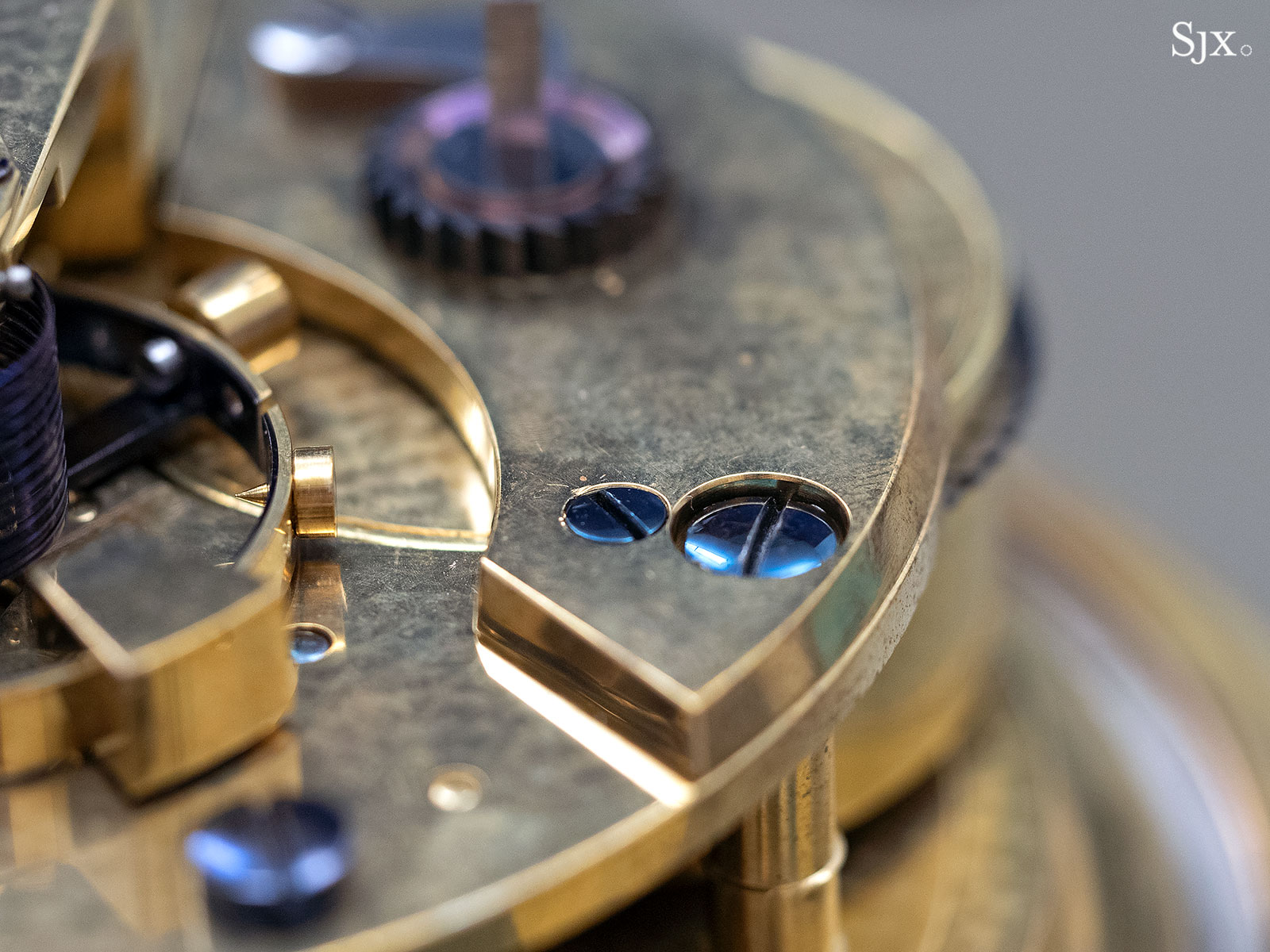
Traditional blued screws provide colour contrast against the brass plates and bridges
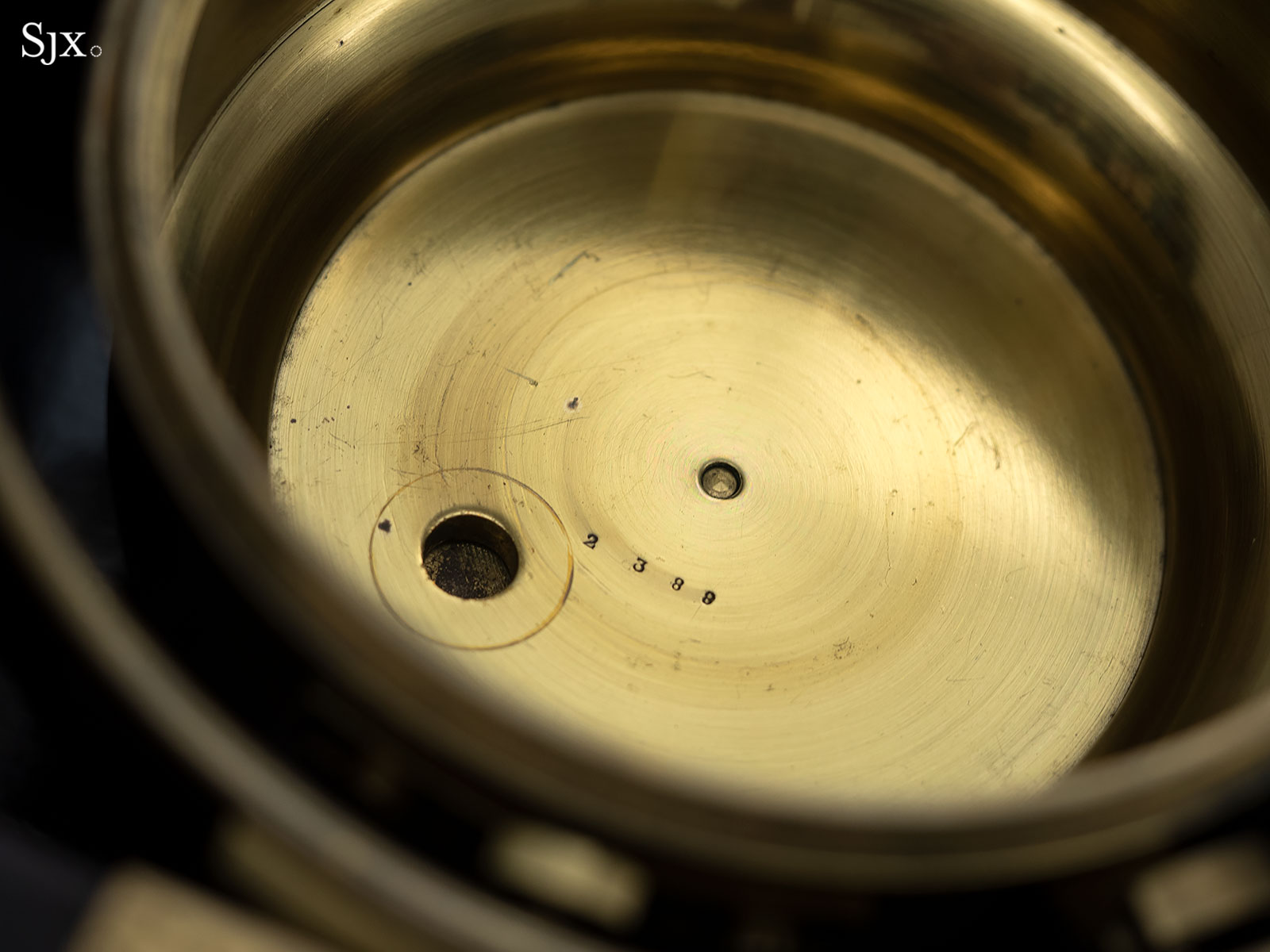
The finely made brass case of the marine chronometer that’s also stamped with the serial number
Technical criticisms
While the idea of an intricate compensating weight is interesting, it does raise a couple of questions about its practicality and real-world function.
The first observation is that it is peculiar to see only one side of the balance wheel equipped with Barraud’s weight, while the other end uses a regular wedge weight. As a result, the shifting mass within Barraud’s weight is not compensated equally on the opposite side, which may result in minor poising variations. Nevertheless, marine chronometers are designed to always remain in an ideal horizontal position thanks to the standard gimbal mounting, which minimised the effect of poising errors.
Another inherent problem is that due to the mechanism contained within, it was not possible to slot Barraud’s weight into the same plane as the balance wheel, unlike the conventional wedge weight. In other words, Barraud’s weight sits on and over the rim of the balance wheel. The consequence is another poising issue and potentially a lopsided force acting on the balance staff – since one side of the balance wheel is heavier – that could affect the balance wheel’s performance.
In theory, to address both these issues, Barraud’s weight should have been installed as a pair to address the poising issue, resulting in an ideally balanced rim.
There is also a question of practicality. Contemporary literature implied the mechanism being finnicky to construct and adjust, which meant that production numbers were likely very low. In fact, this marine chronometer with Barraud’s weight is probably one of just three that still exist in functional condition. The other example, No. 2627, belongs to the National Maritime Museum in London, while No. 2655 belongs to a private collector.
With marine chronometers being generally robust objects, the scarcity of surviving examples indicate that other inventions were more successful and widespread in addressing the middle temperature error, most notably the Guillaume balance.
Ultimately, there are no detailed records of the performance of Barraud’s weight, making it impossible to ascertain if the weight truly worked as intended and offered a superior performance over the standard marine chronometer. The sum of probabilities indicate it was either unsuccessful, or too expensive to be practical, explaining why so few are in existence today.
Technological progress
However, whether Barraud’s weight was an effective solution is not the point. The very concept is interesting, and offers a glimpse at some of the progress charted by horologists in solving important problems in the past. Every little success or failure contributes towards a better future that we take for granted today, such as the Nivarox hairsprings.
And so the little-known success of Barraud’s esoteric invention should be appreciated. At the least, when I next gaze at the stars in a clear night’s sky, I am reminded that those were the exact same celestial landmarks relied upon for millennia to take us where we want to go.
Correction August 22, 2020: The bimetallic balance wheel was invented by Pierre Le Roy, and not John Harrison as stated in an earlier version of the article. Instead, Harrison invented the bimetallic curb for the hairspring.
Update October 24, 2023: New information indicates that there are three known working examples, instead of two.
Back to top.